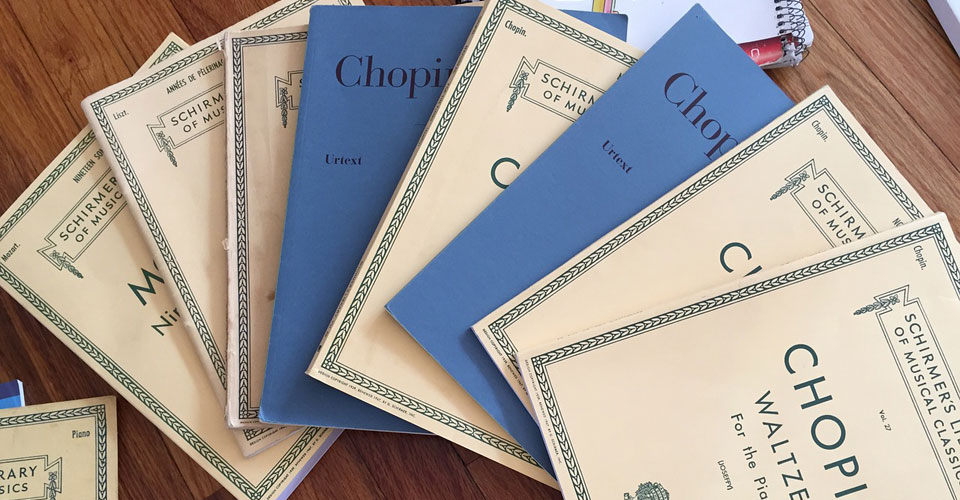
Author: Minna Huotilainen.
Abstract
Recent developments in brain research methodology allow the use of neuroscientific methods in natural learning situations in order to monitor learning while it happens, which makes neuroscience a relevant tool for educational sciences.
The paper discusses the role of neuroscience in understanding learning, shows how the variations in the learner’s physiological status can be measured, and discusses their effect on learning.
Two important lines of neuroscientific research in education are discussed in more detail. First, the benefits of using music in learning are presented from the brain development and plasticity point of view. Second, studies on the use of embodied learning methods are presented, highlighting the role of physical activity, craft and design activities in developing embodied cognitive capacities.
Finally, future trends of neuroscience in learning are presented, drafting a future where neuroscience has an empowering role in the everyday lives of learners. Understanding individual learning and physiological states may change the way that we organize learning.
Introduction
The decade of the brain, the 1990’s, was the advent of neuroscientific methodologies in a large scale. Funding invested in the development of accurate and usable, non-invasive and precise neuroscientific measurement instruments, devices, systems, and methodologies paid off, and multi-channel electroencephalogram (EEG) measurements, magnetoencephalography (MEG) with whole-head systems, structural and functional magnetic resonance imaging (MRI, fMRI) and navigated transcranial magnetic brain stimulation (NBS and TMS) became possible in hundreds and later in thousands of research laboratories around the world.
After the decade of the brain, the neuroscientific devices have taken two opposite paths of development. First, laboratories are investing in more and more accurate devices, including higher magnetic field strengths in MRI and fMRI, very large number of electrodes in EEG and hundreds of channels in MEG, and combinations of adjacent or even simultaneous measurements with different methods. This high-precision line of development makes the results far greater in temporal and/or spatial accuracy and can thus reveal new details of brain structure and function. It should be noted, however, that this development is restricted solely to laboratory settings. For this reason, the second path of device and method development has taken the opposite turn. With a very fast preparation, mobile and affordable devices based often on EEG or other physiological electric signal recorded from skin surface with electrodes or light, offer the possibility to move to the locations of natural environments, schools, day-care centers, work places or even outside, and study real-life events. With this natural-environment path, the temporal and spatial accuracy of the experiment usually cannot be as good as in the laboratory setting, but this is compensated by the natural environment, natural stimulation, and larger amounts of data collected easily from large groups of people even simultaneously and for extended periods of time, sometimes even by the participants themselves.
Both of these development paths, extreme accuracy in laboratory settings and maximal naturalness in real-life settings, are important for learning research. In laboratory settings, the brain indices of key cognitive processes such as perception, attention, memory, and learning can be accurately studied in isolation and basing the studies on the large amount of prior data collected from the same processes in earlier research. Such studies can reveal phenomena that could not be observed without accurate neuroscientific data. An example of studies in both paths, with high relevance for understanding learning, are presented here.
A good example of laboratory-based studies crucial for understanding learning is that of Näätänen et al. (1993). They studied the process of learning to perceive a complex sound (fast mini-melody) and to detect minor changes in the sound (Näätänen et al., 1993). In the first experiment, they gave their fourteen participants a test of detection accuracy asking them to press the button every time they observed a change in the sound. The test was performed three times after being passively exposed to the sounds for 30, 60, and 90 minutes. Five participants could perform the task adequately, while two participants could not detect the changes in the sounds. Interestingly, seven participants learned to detect the changes during the experiment (their performance in detection improved from the beginning to the end of the experiment). In their second experiment, they tested fourteen new participants only once after being passively exposed to the sounds for 90 minutes.
This laboratory-based experiment yielded several interesting and important results. First, the brain responses of the three groups of performers in the first experiment differed drastically from each other. Those five participants who could perform the task already in the initial test showed fast and clear brain responses to the changes, while the brain responses of those who learned to detect the changes improved step by step. Importantly, their brain responses showed increases prior to the learning being evident in their performance, highlighting the possibilities of neuroscience to detect skills that have not yet materialized in the behavior of the learner. Further, no improvement was seen in the brain responses of the participants of the second experiment which involved only passive exposure, highlighting the importance of testing and the power of attentive listening in learning.
A good example of maximal naturalness in real-life learning situations is that of Leinikka et al. (2016). We studied the physiological responses of artists and art students while they were drawing and forming clay in a studio. Here, data from 30 participants were collected during the actual process of drawing and forming, while wearing portable sensors (Faros, Mega Electronics, Finland) capable of recording the full electrocardiogram and accelerometers (Actigraph, GENEActiv, Finland) on their both wrists for hand movement detection. In a quasi-controlled situation, the participants performed three different given tasks (copying based on a photo of a cup, designing a cup, and free improvisation). The cardiac activity measures, especially those reflecting the activity of the autonomous nervous system, showed interesting connections to both the material and to the task that the participants were performing. Working with the clay was physically more demanding than drawing, which was reflected in several heart-rate variability (HRV) parameters. The greatest amount of free mental resources was observed in the design and improvisation tasks in fast drawing compared to any other task. The results suggest that free improvisation involving drawing fast, improvised works, seems to be the most effective way of freeing mental resources. The HRV effects were consistent with the participants’ own views of physical and mental stress. This study shows that even demanding learning tasks related to creative work can be studied with physiological methods in conditions that greatly resemble natural learning situations.
The two experiments above are presented in order to highlight the possibilities of neuroscientific and physiological measurements in understanding learning processes. A good knowledge of the brain indices of memory, attention and perception in laboratory-based settings allows researchers to move away from laboratories and to study learning in real-life situations. Results from physiological studies highlight the importance of building motivation, states of flow, collaboration, and goal-directed actions in learning, and the importance of the awareness of the physiological state of the learner (see below). Such studies also show the harmfulness of poor learning environments, including acoustic and visual noise, for learning (see below). For the first time in history, we now have the tools to monitor learning while it happens, and to see how the brain reacts to different types of learning environments, learning methods, and physiological conditions. This makes neuroscience a relevant tool for educational sciences.
Below I will present some examples and the most relevant approaches from neurosciences to education and learning.
Neuroscience of learning environments
Learning environments have important physical and mental characteristics that give boundary conditions for learning. Studying distractions caused by sudden sounds in the environment have shown that surprising, unpredictable sounds make learning less efficient. Every time a sound starts in the environment, even when the learner is not paying attention to it or does not feel distracted by the sound, the auditory system will invest some resources in analyzing the sound acoustic properties. These analysis processes are partially unconscious and have developed in order for the auditory system to be able to detect potential dangers in the environment.
The most distracting background sound stimulus in learning situations is intelligible speech. Speech that can be understood will load the phonetic loop of the brain, which is also needed in reading, writing, and speaking. In contrast, unintelligible babble does not load the phonetic loop.
Interestingly, listening to background music during learning may be beneficial for some learners. In most studies, listening to fast and pleasant music prior to learning has been shown to enhance learning and performance in cognitive tests, while results from studies researching the effects of listening to music while learning are more mixed.
Acoustic characteristics of learning spaces affect learning. Long reverberation times and high background noise levels decrease speech intelligibility and increase the effort needed to achieve learning.
Also visual noise in learning environments can be disturbing to learning. Observing movement especially in the peripheral areas of the visual field gives rise to distractions. Such problems are far fewer in smaller rooms and smaller group sizes.
The key importance of the physiological state for learning
The physiological state can be defined as a combination of the operating modes of the physiological systems in the human body, including the autonomous and central nervous systems and especially the hypothalamus-pituitary-axis, the hormonal systems, the cardiac and blood circulation systems, breathing, and muscle movements including blinking. Most typically, these systems follow the emotional state and physical activity of the individual, optimizing the mental and physical performance for each condition.
The cardiac system is a fast-reacting system that reflects the presence of both physical stressors like movement and physical activity, and mental stressors like fear or anxiety. The activity of the cardiac system is most typically investigated by recording the electro-cardiogram (ECG), an electric signal originating from the heart muscle and nerves that accurately depicts the fast and slow regulatory changes in the activity of the heart. For example, the heart-rate variability (HRV) is the natural fluctuation in the beat-to-beat (R-R) intervals of the heart. It reflects the current activity status of the sympathetic and parasympathetic autonomous nervous system. Increased mental stress leads to decreased HRV, which is a sign of the activation of the sympathetic nervous system and a decrease in the activity of the parasympathetic nervous system. This is typically associated with faster heart rate, higher muscular tension, faster breathing, and feeling tense, irritated and/or restless. Generally, short-term increase in HRV is associated with relaxation, openness and positive mental efforts, while long-term high levels of HRV are related to good health. Previous studies (Cinaz et al., 2013) have shown that several time- and frequency-domain HRV measures are relevant in tracking the interaction between cognitive and physiological processes. It should be noted that while levels of physiological arousal and stress are highly correlated with these physiological signals, there is no measure of the direction of the emotional valence, i.e. is the learner experiencing positive or negative emotions.
The variations in the learner’s physiological status are highly important in characterizing the learning. States of flow are the most optimal physiological states of learning, and feelings of fear, threat or other strong negative emotions, as well as low-activity negative emotions like boredom are not boosting or enabling learning. It is important to note that these can be measured and recognized, both in individual learners and in groups of learners, with non-intrusive, low-cost devices, and this information can be used to guide learning. Specifically, choosing the learning methods, environments, groups, goals and tasks of learning could be directly based on the physiological data of the learners. Such data could be empowering to the learners and guide them in understanding and optimizing their own learning.
Maestro, music, please! How could music enhance learning?
The benefits of using music in learning have been shown both from the brain development and plasticity point of view, showing benefits of long-term practicing to play a musical instrument or to sing, but also in terms of short-term benefits altering one’s physiological state by listening to carefully chosen music.
Several studies have shown the long-term benefits of learning music: the musician’s brain in terms of cortical and subcortical capacity, connections, both in structure and function, are used as an example of learning benefits of music activities. Adult musicians’ brains show changes that seem beneficial both in young and old age. Long-term cognitive benefits of music learning have been shown in small children (Hyde et al., 2009; Putkinen et al., 2014a), school-aged children (Putkinen et al., 2014b), and hearing-impaired children (Torppa et al., 2014a, 2014b). Minor deficiencies in language processing have been shown to be alleviated with group music activities (Kraus et al., 2015; Overy et al., 2000; 2003). All in all, learning to sing and play a musical instrument has plastic effects on the brain that make it easier to learn other things.
Music is very fast in changing the physiological state. Already after some dozens of seconds of listening to music, physiological changes in bodily functions can be measured. Thus, carefully chosen music is a potential tool for changing the physiological state towards a more optimal state for learning. Many learners practically know how to use music to help their learning: they listen to their favorite, fast, energizing music before doing their homework to motivate themselves, or reduce stress by listening to calming, pleasant music (Saarikallio et al., 2013). Conscious use of music prior to or even during learning may help some learners, but it can also be distracting. It is important to note that the effects of music vary largely across individuals according to their distractability and history of music listening. Individuals with musical training are more often distracted by background music during learning than others, while individuals with attentional problems seem to benefit from background music more often than other learners.
Embodied learning occurs in the brain with the help of the body
Embodied cognition can be defined as a dependency and shaping of human cognition by its interaction with its environment by using the body (Johnson, 1987; 2007; Lakoff & Johnson, 1999; Noë, 2004; 2009). Action and perception appear as key methods in knowledge formation, creativity, and learning. Hence, neuroscientific research related to embodiment and motor activities demonstrate how motor processes are connected to cognitive functions (Borghia & Cimattic, 2010; Rizzolatti & Craighero, 2004). Embodied cognition is reflected in the research paradigms and research designs of cognitive science, when researchers study the human-environment system as well as the situated and embodied nature of human cognition (Hari & Kujala, 2009).
Craft and design activities are complex brain activities that allow part of our cognitive capacities to be located in an embodied space, between the brain and the hands, and between brains and hands of groups of people. Utilizing craft and design as learning methods is making use of embodied capabilities of the learners.
Physical activity during learning and as a lifestyle have been shown to affect learning capabilities and especially memory functions. Interventions of physical activity are shown to increase learning results both in school children and in the elderly. Increase of physical activity was also shown to increase the volume of the hippocampus and enhance memory functions.
Several neuroscientific studies presented above and elsewhere reflect the importance of the active use of the body, in crafts, in design, and in physical activity in general, for the optimisation of learning. The applications of this knowledge, however, are not straightforward and need pedagogical development in order to succeed. Potential caveats include auditory and visual distraction when physical movement is not relevant or guided.
Future use of neuroscience in learning and education
The future use of neuroscience in understanding learning include research at several levels. First, highly accurate simulations of learning events, occurring in brain research laboratories with precision instruments, offer new insight into the details of learning processes. Second, experiments in natural conditions in schools, day-care centers, universities and work places will help us understand a holistic view of learning, taking into account different learning methods, learning spaces and inter-individual differences in a much more detailed fashion than ever before. In the future, it is possible that schools want to utilize such measurements when planning large-scale changes like changes of learning environments, or when aiming at solving problems in learning. Third, neuroscientific measurements may become a normal part of the daily lives of learners. We can compare this development to the treatment of diabetes: currently, the measurement of blood sugar level is something that diabetic individuals do every day, even though some decades ago it was not possible to get such information so quickly. In the future, each learner may want to have information on his/her brain activity, physiological status, and learning in order to optimize learning efforts and outcomes. Solutions for making the measurement easy and the data analysis automatic are becoming more and more efficient. It is just a matter of time when we have such devices and possibilities for every learner.
Understanding individual learning and physiological states may change the way that we think about learning and how we organize it. This information may profoundly change the way that we understand what learning actually is. Such changes will also demand large-scale changes in schools and in education, in their environments and how the whole educational system is organized.
Author
Minna Huotilainen, D.Sc., Professor of Educational Sciences, University of Helsinki, Finland
[vc_tta_accordion active_section=”0″ no_fill=”true” el_class=”lahteet”][vc_tta_section title=”References” tab_id=”1458134585005-b3f22396-5506″]
Borghia, M. & Cimattic, F. (2010) Embodied cognition and beyond, Neuropsychologia, 48, pp. 763–773.
Cinaz, B., La Marca, R., Arnrich, B., & Tröster, G. (2010). Monitoring of mental workload levels, In Proceedings of IADIS eHealth conference.
Hari, R & Kujala M. M.(2009). Brain Basis of Human Social Interaction: From Concepts to Brain Imaging, Physiological Reviews. Vol 89, pp. 453–479.
Hyde, K. L., Lerch, J., Norton, A., Forgeard, M., Winner, E., Evans, A. C., & Schlaug, G. (2009). Musical training shapes structural brain development. The Journal of Neuroscience, 29(10), 3019–3025. https://doi.org/10.1523/JNEUROSCI.5118-08.2009
Johnson, M. (1987). The body in the mind, Chicago: Chicago University Press.
Johnson, M. (2007). The meaning of the body, Chicago: Chicago University Press.
Kraus, N. & Strait, D.L. (2015). Emergence of biological markers of musicianship with school-based music instruction. Annals of the New York Academy of Sciences, 1337, 163–169.
Lakoff, G. & Johnson, M. (1999). Philosophy in the flesh: The embodied mind and its challenge to western thought, New York: Basic Books.
Leinikka, M., Huotilainen, M., Seitamaa-Hakkarainen, P., Groth, C., Rankanen, M., & Mäkelä, M. (2016). Physiological measurements of drawing and forming activities. Proceedings of DRS2016: Design+ Research+ Society-Future-Focused Thinking.
Näätänen, R., Schröger, E., Karakas, S., Tervaniemi, M., & Paavilainen, P. (1993). Development of a memory trace for a complex sound in the human brain. Neuroreport: An International Journal for the Rapid Communication of Research in Neuroscience.
Noë, A. (2004). Action in Perception. Cambridge: The MIT press.
Noë, A. (2009). Out of our heads, New York: Hill and Wang.
Overy, K. (2000). Dyslexia, temporal processing and music: The potential of music as an early learning aid for dyslexic children. Psychology of music, 28(2), 218–229.
Overy, K. (2003). Dyslexia and music. Annals of the New York Academy of Sciences, 999(1), 497–505.
Putkinen, V., Tervaniemi, M., Saarikivi, K., de Vent, N., & Huotilainen, M. (2014). Investigating the effects of musical training on functional brain development with a novel Melodic MMN paradigm. Neurobiology of Learning and Memory, 110, 8–15. https://doi.org/10.1016/j.nlm.2014.01.007
Putkinen, V., Tervaniemi, M., Saarikivi, K., Ojala, P., & Huotilainen, M. (2014). Enhanced development of auditory change detection in musically trained school-aged children: a longitudinal event-related potential study. Developmental Science, 17(2), 282–297. https://doi.org/10.1111/desc.12109
Putkinen, V., Saarikivi, K., Tervaniemi, M. (2013). Do informal musical activities shape auditory skill development in preschool-age children? Frontiers in Psychology, 4, 572. doi: 10.3389/fpsyg.2013.00572
Rizzolatti, G., & Craighero, L. (2004). The mirror-neuron system. Annual Reviews of Neuroscience, 27: 169–192.
Saarikallio, S., Nieminen, S., & Brattico, E. (2013). Affective reactions to musical stimuli reflect emotional use of music in everyday life. Musicae Scientiae, 17(1), 27–39.
Torppa, R., Faulkner, A., Huotilainen, M., Järvikivi, J., Lipsanen, J., Laasonen, M., & Vainio, M. (2014a). The perception of prosody and associated auditory cues in early-implanted children: the role of auditory working memory and musical activities. International Journal of Audiology, 53(3), 182–191.
Torppa, R., Huotilainen, M., Leminen, M., Lipsanen, J., & Tervaniemi, M. (2014b). Interplay between singing and cortical processing of music: a longitudinal study in children with cochlear implants. Frontiers in Psychology, 5, 1389.
[/vc_tta_section][/vc_tta_accordion]